TECHNOLOGY FOCUS
The course content will consist of steering and focusing antenna beams using plasma physics, VSWR, S11, radiation patterns, applications to MRI/PET, pulsing circuitry for ionizing plasma antennas, surfatrons, surface wave excitation, plasma metamaterial antennas and plasma metamaterial frequency selective surfaces, atmospheric plasma antennas, plasma resonances on plasma dipole antennas, progress on ruggedization of plasma antennas, and FAR-UVC to kill covid-19 and other viruses.
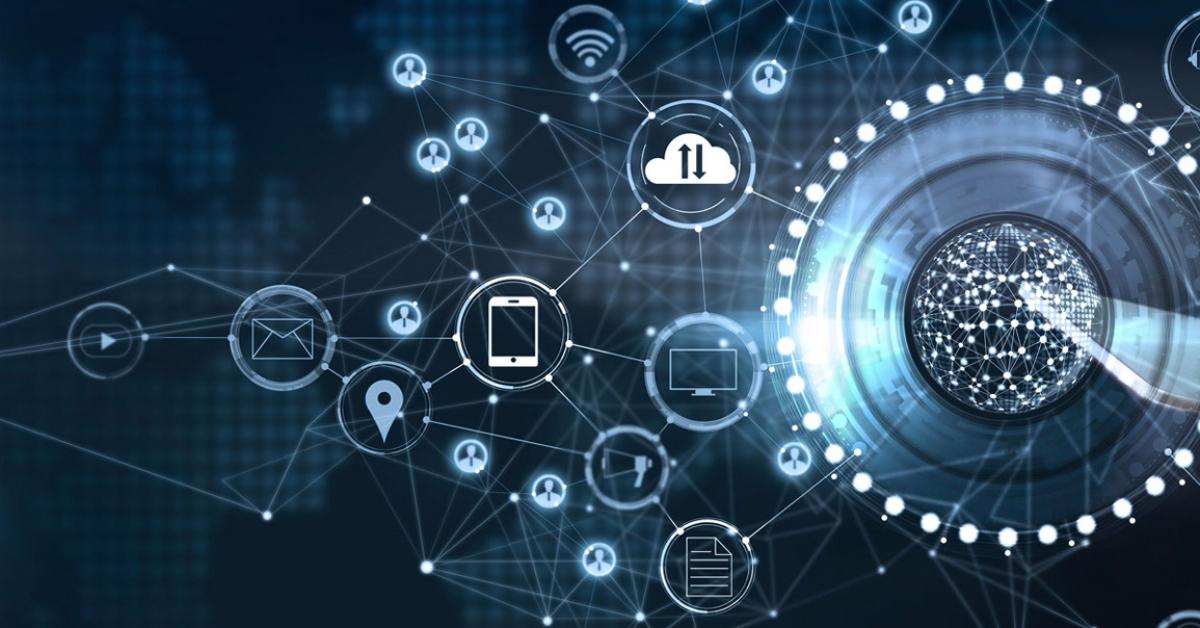
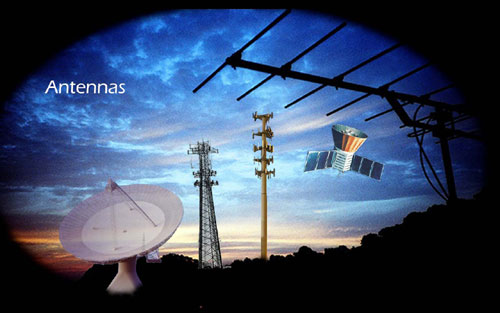
COURSE CONTENT
The course content will consist of steering and focusing antenna beams using plasma physics, VSWR, S11, radiation patterns, applications to MRI/PET, pulsing circuitry for ionizing plasma antennas, surfatrons, surface wave excitation, plasma metamaterial antennas and plasma metamaterial frequency selective surfaces, atmospheric plasma antennas, plasma resonances on plasma dipole antennas, progress on ruggedization of plasma antennas, and FAR-UVC to kill covid-19 and other viruses.
WHO SHOULD ATTEND
The people who should attend should have taken the first course on plasma antennas and have a BS degree or European Diploma in physics or electrical engineering. No background in antennas or plasma physics is required. Basic electromagnetics, antenna theory, and plasma physics will be taught.
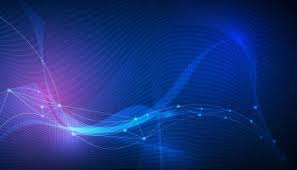
Day 1
13. Steering, Focusing, and Spreading of Antenna Beams using the Physics of Refraction of EM Waves through a Plasma.
13.1 Introduction
13.2 Basic Physics of Refraction Theory of Electromagnetic Waves Propagating Through a Plasma.
13.3 Antenna Beam Focusing from Refraction through a Plasma Experiments and Simulations.
13.3.1 Peak Current vs Average Current Due to Pulsing to Ionize the Gas into a Plasma.
13.3.2 Experiments on Focusing Antenna Beams with The Physics of Refraction through a Plasma.
13.3.3. Simulation of Plasma Focusing by Refraction Through a Plasma.
13.3.4. Three D Simulation of Plasma Focusing by Refraction Through a Plasma with 10 GHz plasma frequency and 24 GHz incident frequency.
13.4. Antenna Beam Steering with Refraction through a Plasma.
13.4.1. Experiment with Steering from Refraction through a Plasma with 5 Amp and 8 Amp Peak Current in Pulsing.
13.4.2. Experiment with Steering from Refraction through a Plasma with 5 Amp and 8 Amp Peak Current in Pulsing.
13.4.3 Simulations of Steering Antenna Beams by Refraction through the Plasma with Incident Frequency of 44 GHz and Various Plasma Frequencies.
13.4.4. Experiment with Steering from Refraction through a Plasma with 5 Amp and 8 Amp Peak Current in Pulsing.
13.4.5 Simulations of Steering Antenna Beams by Refraction through the Plasma with Frequencies of 35 GHz to 45 GHz and Plasma Frequency Fixed at 22.9 GHz.
13.4.6. Experiment with Steering from Refraction through a Plasma with 0 Amp and 8 Amp Peak Current in Pulsing.
13.4.7. Simulation with Steering from Refraction through a Plasma with 0 Amp and 8 Amp Peak Current in Pulsing. Plasma Frequency 20 GHz and Incident Frequency 44 GHz.
13.4.8 Three D Simulation with Steering from Refraction through a Plasma with 8 Amp Peak Current in Pulsing. Plasma Frequency 20 GHz and Incident Frequency 44 GHz.
13.5 Simulations of Antenna Beam Steering by Refraction through a Plasma with Variations in Plasma Frequency with Main Lobe and Side Lobe Characteristics.
13.6 Basic Plasma Beam Steering Device.
13.7. Antenna Beam Spreading by Refraction of EM Waves through a Plasma.
13.8. Summary of Using Plasma to Focus, Steer, and Spread Antenna Beams.
Day 2
14. Pulsing Circuitry for Ionizing Plasma Antennas with Low Power and High Plasma Density Requirements and Surface Wave Excitation with Surfatrons.
14.1 Pulsing circuit to ionize the plasma with high plasma density and low power.
14.2. High voltage pulse forming network (PFN) for faster and more efficient pulse generation.
14.3 Ionization of the Gas into a Plasma by Surface Waves
14.3.1. Introduction to Surface Wave Ionization with Surfatrons.
14.3.3. Plasma Inverted F Antenna Tunable with Reconfigurable Length and with Surface Wave Ionization with a Surfatron.
14.3.4. Hybrid Plasma Antennas with Surface Wave Ionization with Surfatrons.
15. Radiation Patterns, S11, and VSWR of the Smart Plasma Antenna.
15.1 Introduction
15.2 Basic Smart Plasma Antenna Design
15. 2.1. Typical characteristic plasma values in a COTS (commercial off the shelf) tube used as a plasma antenna.
15.3 Experimental Setup of Smart Plasma Antenna Measurements.
15.3.1 Smart Plasma Antenna Tube Configurations in which Radiation Patterns were Measured.
15.4 Resonance Frequency of the Smart Plasma Antenna
15.5 Measurements of S11 and VSWR.
15.6 Smart Plasma Antenna Radiation Patterns.
15.6.1 Radiation Pattern Measurement in an Open Field.
15.6.2 Radiation Pattern Measurements in a Satimo Chamber.
15.6.3 Directivity of the Smart Plasma Antenna
15.7 Simulations on the Smart Plasma Antenna with One Tube Off.
15.8. VSWR measurements on the first and fundamental resonance of the smart plasma antenna.
15.9 Future Design Improvements to Increase Gain.
15.10. Wi-Fi Estimations of the Smart Plasma Antenna.
15.11 Applications to 5 G and Cellular in General.
15.12. Plasma Antenna with Variable Magnetic Field and Plasma Density.
Day 3.
16. Applications of Plasma Antennas to MRI/PET.
16.1 Introduction.
16.1 The Problem with Metal RF Coils in an MRI Machine.
16.2 Basic Plasma Antenna used in place of Metal RF Coils.
16.3 Plasma Ignition in a Strong Magnetic Field
16.4. Ionizing the Gas with Surface Waves and the Surfatron Matching Circuits.
16.5. Imaging Experiments with Basic Plasma Antennas.
16.6. Positron Emission Tomography with Plasma Antenna RF Coils.
17. Experiments on Co-site Interference, VSWR, and Noise of Plasma Antennas.
17.1 Introduction
17.2 Co-site Interference.
17.3 VSWR.
17.4 Experimental Measurements of Noise.
17.5 Part 2 Experiments of Co-site Interference and VSWR
17.5.1 Impedance Matching
17.5.2 30-88 MHz Plasma Antenna
17.5.3 116-174 MHz VHF Band
17.6 Part 3. Experiments of Co-site Interference and VSWR.
18. Plasma Metamaterial Antennas and Plasma Metamaterial Frequency Selective Surfaces, Atmospheric Plasma Antennas, Plasma Resonances on Plasma Dipole Antennas, and Progress on Ruggedization of Plasma Antennas.
18.1 Plasma Metamaterials and Plasma Photonic Band Gaps for Plasma Antennas and Plasma Frequency Selective Surfaces.
18.2 Experiment in Scattering Electromagnetic Waves off of Metal Photonic Crystal with a Metal Tube Replaced by a Plasma Column.
18.3 Atmospheric Plasma Antennas.
18.4 Plasma Resonances on a Cylindrical Plasma.
18.4.1 Experiments and simulations on plasma resonances of a cylindrical plasma column.
18.4.2 Simulations and experiments of resonances in a plasma dipole antenna with a 100 MHz to 5 GHz sweep.
18.4.3 Understanding some characteristics of the plasma by pulsing the plasma and observing the plasma recombination or decay of a cylindrical plasma column.
18.4.4. Simulations on a plasma dipole antenna as a function of density and gas type.
18.4.5. Electrically Small Monopole Antennas using Plasma Physics
18.5. Minimum Ionization Current to Create a Plasma Antenna
18.6. Pre-ionization Current to Make Ionization faster and with Less Power
18.7. Progress on Ruggedization on Plasma Antennas.
18.8. FAR-UVC to kill covid-19 and other viruses.
Dr. Anderson has published texts that are a recommended reference. The references are not required for this course.
Plasma Antennas, Second Edition, (Artech House, 2010) ISBN: 9781630817503
My original book titled “Plasma Antennas”, (Artech House, 2010) ISBN: 78-1-60807-143-2