TECHNOLOGY FOCUS
Although RF circuits are generally considered to be circuits that operate from tens of MHz up to several GHz, and Microwave circuits at frequencies beyond that, boundaries based purely on frequency are rarely appropriate. Analog integrated circuits based on lower-frequency design methodologies can now operate well into the microwave range, purely because of smaller feature sizes that are available in CMOS and silicon-germanium technologies. Integrated circuits that operate in the microwave frequency range, designed using low frequency architectures, are now abundant.
However, don’t think that the art of circuit design is no longer needed because of the ready availability of drop-in building blocks that do it for you! Using ready-made IC chips still requires matching, tuning, bias and stabilisation networks that must be modelled and designed.
Classical high frequency microwave circuit design techniques are still relevant and important to engineers even if they never need to build a bespoke circuit from scratch themselves. It is still critical to be able to model and understand problems arising from noise, mismatch, circuit losses, and limited bandwidth in integrated building blocks and the matching elements and filters that surround them.
We will focus on the design of discrete circuits that are differentiated from their historically lower-frequency counterparts by several features. In RF and microwave design, the phase shift of the component is significant because its size is comparable with a wavelength, its reactances and parasitics must be accounted for, and reflections occur between elements. These all limit bandwidth. We also need to consider circuit losses that degrade the Q of an element as well as introduce noise, and nonlinearities that introduce distortion into the signal path. Electromagnetic radiation and capacitive coupling will also be features of such circuits. Such 'RF and microwave' effects are most commonly observed when using discrete or custom devices, or when assembling integrated circuits together at higher frequencies into systems. Ignorance of such effects can result in reduced bandwidth, unwanted spurious signals, elevated noise, unexpected oscillation, or degraded linearity.
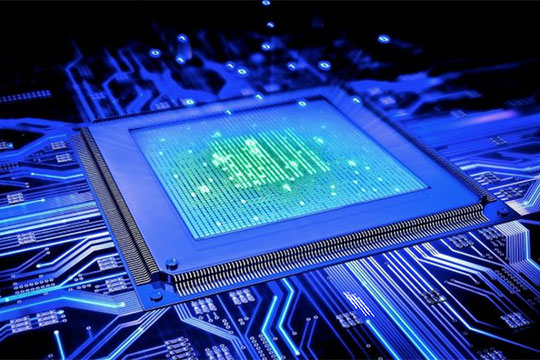
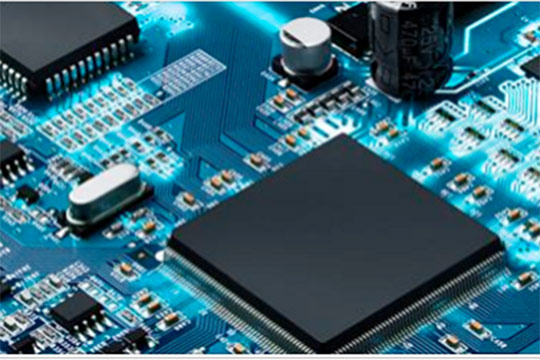
COURSE CONTENT
While focusing on the design of discrete RF and Microwave circuits to show classical microwave design techniques, examples of system integrated on chip are presented to compare the 'two worlds'. As CMOS design now extends even beyond Ka-band frequencies, many classical microwave design insights and techniques are being forgotten. For instance, the use of a source inductor in a CMOS low-noise amplifier stage is widespread for simultaneously improving gain and noise match, but why? This course gives a renewed understanding of why and how such tricks are effective.
Impedance matching, circuit stability, power output, distortion, power combining, and component losses and parasitics are all examined in the context of microwave circuits in modern systems. We will also explore device modelling using state-of-the-art low-voltage transistors so we can reconcile small signal and large signal design techniques. This is illustrated in a number of applications such as small-signal, large-signal, low-noise, and feedback amplifiers with discrete transistors. Low-noise design considerations are also introduced, using CAD modelling of reactive and resistive types of applications. Reflecting its importance as a fundamental building block of most systems, and as an opportunity to demonstrate computer modelling of most other circuit types, amplifier design is treated exhaustively, using both small signal S-parameter methodologies (for noise, gain, and matching) and large signal models (for power and distortion).
Oscillators and mixers are also designed to meet demanding systems requirements. The course first explains the fundamental operating principles of these components in great simplicity, and then illustrates the theory elegantly through practice. We show the importance of modeling parasitic elements that arise in design or when interconnecting components at high frequencies. We also consider oscillator and mixer performance using a system simulator, reviewing how these components need to be specified for use in communication systems, for instance to maintain I and Q channel orthogonality, and how this relates to the system performance. We will also look at higher level RF and microwave subsystems, such as LNBs and BUCs.
Nonlinear design techniques are also examined with a harmonic balance simulator, using bipolar, FET, and HEMT devices. The course emphasises hands-on design and simulation of many circuit types, considering their linearity, efficiency, and power requirements. We develop an intuitive understanding of how non-linearity arises, and its impact on design, together with more detailed circuit modeling to examine quantitative impact.
To benefit most, bring your own laptop computer, and prior to attending the course, obtain a free trial license of Microwave Office (MWO) from AWR at www.awrcorp.com .
On completion of the course, you will be able to:
- Understand matching circuits and topologies to create the most important elemental building blocks of systems, using single transistors or fully integrated chip sets
- Design small-signal, low-noise, and power amplifiers
- Perform RF stability analysis and eliminate potential instabilities
- Design oscillators and VCOs for low-phase noise or output power
- Design mixers using FETs, bipolars, and diodes
- Understand the power of microwave CAD tools, achieve better first-pass design success, and be better placed to troubleshoot problems when circuits don´t work as expected.
WHO SHOULD ATTEND
This course is most helpful for engineers with some understanding of electronic circuits already, but who may be wishing to move into microwave or RF circuit design – either active or passive.
The course is suitable for engineering managers, design engineers, and experienced test and production engineers.
Past participants have benefitted most from gaining a more thorough and systematic approach to the design and use of microwave circuits. They typically work in companies designing microwave components or subsystems, in defence, radar, communications, or in the emerging ‘new space’ and Internet-of-Things industries.
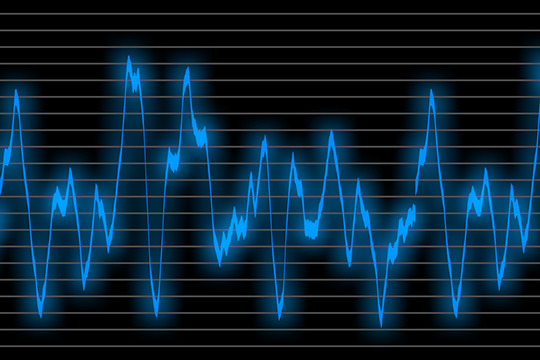
Day 1
Classical small-signal RF Circuit Design
To introduce linear active circuits, we review the fundamental principles of impedance matching and move on to examine the effect of mismatch on gain, circuit stability and power performance.
- Revision of S-parameters, Matching and the Smith Chart
- Gain in a distributed circut - impedance transformation
- Unilateral Gain Circles in Small-signal Amplifier Design
- Complex conjugate matching for maximum gain
- RF Circuit Stability: Graphical and analytical techniques
- K- and µ-Factors, Nyquist Stability Analysis
Example: Broadband Transistor Stabilization
- Simultaneous Conjugate Match, Bandwidth Considerations
- GMAX and MSG Definition
Example: 1900MHz Amplifier Design for Maximum Gain
Day 2
Discrete Low-Noise and Broadband Amplifiers
We examine the three commonly used techniques used in maximum small-signal gain, low-noise, and linear power amplifiers.
- Transducer-, Operating-, and Available-Gain Techniques
- RF Noise Sources, Noise Figure and Noise Measure
- Constant-Noise and Constant-Gain Circles in LNA Design
- Available-Gain Design for Minimum Noise
- Trade-Offs Between Gain, Match, and Noise Performance
Example: 900MHz Discrete LNA Design
- Broadband Amplifier Design Techniques
- Reactive Mismatch and Lossy Matching Techniques
- Feedback Amplifiers Combined with Impedance Matching
- Circuit Optimization for Gain, Match and Stability
Example: 1-4000 MHz Feedback Amplifier Design
Day 3
Power Amplifier Design
- Design for Optimal Power - nonlinear circuit analysis
- Quasi-Linear Methods to Achieve Power Matching
- Load Line Characterization
- Load Pull Characterization - Measurement and Prediction
- Classes of Power Amplifiers: A, AB, B, C, and F
- Harmonic Tuning to Optimize Efficiency
Day 4
Power Amplifier Design (cont'd)
- Distortion Reduction Techniques
Example: Bipolar Power Amplifier Design Example (CDMA)
Next, we look at the fundamentals of high Q oscillators, paying particular attention to phase noise and tuning bandwidth.
Low Noise (LC) Oscillators
- Oscillator Design Considerations
- Device - Circuit Interaction (Series and Shunt Resonances)
- Deriving the VCO Tuning Curve
- Phase Noise and its impact on System Performance
- Why a system engineer worries about phase noise
Day 5
Low Noise (LC) Oscillators (cont'd)
Example: Bipolar Transistor (HBT) VCO Design in the 4 GHz Band
Finally, we consider mixers and frequency translation, and by simple consideration of mixing theory, uncover the secret to good mixer design.
Mixer Design
- Revision of Diode Mixers
- Bipolar and MESFET Mixer Analysis
- Comparison of Mixer Types
- The Importance of Quadrature Balance
- Modulators, Image-Reject, and Single Sideband Mixers
Example: FET and Bipolar Transistor Active Mixer Designs
Said about the course from previous participants:
"Broad knowledge of the teacher."
"Computer simulations to illustrate lecture topics."
"Very good mixture of theory, practical information and simulation."